23 January 2023
Local Inertia
The de-commissioning of nuclear power plants (and more generally, all types of conventional thermal power plants) is decreasing the inertia of the Belgian power system.
Context
Inertia is provided by rotating masses (typically, turbines of thermal power plants) and acts as an ‘Energy Buffer’, contributing to the damping and the smoothing of system frequency variations in response to power imbalances in the system. At the moment of writing, the level of inertia in the Continental-European power system is still sufficiently large. Yet, for large, interconnected power systems like Europe, uneven inertia distribution could in the future give rise to power system areas with reduced amounts of local of inertia. If a contingency occurs in these low inertia areas, the local variation of frequency could be such that it triggers (generation) protection schemes or even pose a threat for the operation of thermal power plants in the affected area. To some extent, this can in turn start representing a real, future risk for the power system security, since it could trigger cascading failures that could eventually lead to a black-out. As more and more conventional thermal power plants are replaced by zero-inertia converter-based generation (driven by the integration of renewable energy resources), it becomes urgent for transmission system operators to have analysis tools at their disposal that are able to rapidly detect these low inertia areas, so that they can take preventive counteractions, when required.
Approach
In order to monitor the effects of uneven distributions of inertia in the power system, the power system has to be divided into different inertial zones that are composed of groups of generators that behave in a similar way in response to a severe disturbance in the system (short-circuit or generator tripping, for instance). The standard approach to this consists in simulating the behaviour of the system to multiple disturbances and to use the outcome of these time-domain simulations to group together generators with similar responses. However, practical operational constraints make it impossible to rely on lengthy time-domain simulations, since they are too computationally expensive. Indeed, the definition of inertial zones is impacted to a large extent by the operating conditions and depends heavily on the topology of the power system, which are both changing regularly. Therefore, from an operational point-of-view, it is necessary to be able to rapidly re-generate results in response to any system change. As such, time-domain simulations are not an option to develop tools that allow to quickly assess the system security margins linked to local inertia distributions.
Instead, the proposed approach in this project introduces an advanced algorithm that is able to identify clusters of generators that behave in a similar way in response to large disturbances. It does so by focusing on the electrical lay-out and characteristics of the power system. In relies on a graph representation of the power system and does not require any time-domain simulation results, which facilitates to be re-run easily in order to evaluate the impact of changing operational conditions of the power system. Each node of the graph accounts for a simplified representation of a generator and the weights attributed to each branch of the graph reflect the “connection strength” between these equivalent generators. Indeed, strongly coupled generators will have a higher probability to behave similarly than poorly coupled generators.
Once clusters of generators are identified, RoCoF-based indices assess the risks related to low levels of inertia in each of the resulting cluster. RoCoF stands for Rate of Change of Frequency, in other words, the speed with which the frequency drops or rises. This value can easily be estimated analytically for the resulting clusters, and is directly related to the level of inertia in the cluster, hence directly reflecting potential low inertia security issues. The proposed approach goes even further, as it is able to estimate, for a short amount of time, the local frequency evolution in each cluster in response to the largest possible loss of power. Based on the estimated local evolution of the frequency over time (and hence the local evolution of the RoCoF), it is possible to assess, with a better accuracy, the risks associated to fluctuations in frequency for the various clusters and consider how long certain critical thresholds are surpassed. Note that all these approximative formulations are analytical and do not require time-domain simulations, so that the resulting high-level screening indices can readily be used for fast screening purposes in close-to-real-time operational studies.
The proposed approach is thus a logical first step to precisely locate power system areas with potential issues before e.g. embarking on a more detailed analysis (involving time-domain simulations) for the critical parts of the power system. As an illustration, a flow-chart representation of the proposed approach is shown in Fig. 1.
Even if the main objective of the project has been to develop an innovative approach that can be used in operational studies of the Belgian power system, it also has the potential to support planning decisions or could be integrated in optimal power flow problem to check whether optimal power dispatch plans of generators do not violate “local-inertia constraints”.
Results
First, the approach has been validated on a test system that represents in a simplified manner the Nordic region. Results of the clusterization algorithm are convincing and match in next to all cases with the results obtained through time-domain simulations. Moreover, local frequency evolutions obtained analytically are close to the local frequency evolutions obtained from detailed time-domain simulations of the power system. It has been also checked that the approach is sufficiently conservative in a way that local RoCoF values are always slightly over-estimated, such that the screening methodology does not risk on missing out on potential risks that would require further investigation.
Next, the approach has been applied on a large power system model representative of the Continental-European power system. This is a very large and complex model, such that identification of critical (low inertia) areas through time-domain simulations, for a single configuration of the system, would take multiple days. In comparison, applying the proposed approach to this large-scale model takes less than one minute, which allows to use the proposed approach in close-to-real-time implementations in control rooms.
Results of the proposed approach are in line with sound “engineering expectations” and have been validated against detailed time-domain simulations of the power system. In overall, results show a comfortable security margin regarding local level of inertia in Continental Europe at the time of writing. However, in a future where conventional generation is phased out in exchange for converter-based generation (e.g. wind and solar), locational aspects of system inertia will have to screened closely in order to identify potential local problems and propose adequate solutions.
Partners
![]() |
![]() |
![]() |
![]() |
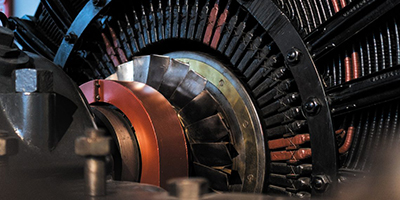
